Global aviation and its emissions contribute to anthropogenic impact on climate. Carbon dioxide (CO2) has a long-term effect on climate over several decades, with the consequence that the CO2 greenhouse effect is independent of daytime, altitude and weather situation. Unlike non-CO2 emission’s climate impact, CO2 emission is highly dependent of the underlying atmospheric conditions, so its forcing depends on location and time of emission. Direct effects result from radiative forcing by emitted greenhouse gases CO2, water vapour and emitted particles. As secondary effects, emitted nitroxides react chemically and affect the concentration of the greenhouse gases ozone and methane. Furthermore, emitted particles favour the formation of contrail-induced cirrus clouds.
In the course of the ClimOP project, the contributing researchers have been investigating the effects of the two operational concepts: Climate-optimised Intermediate Stop Operations and flying low-and-slow. Clim-OP assessed aviation’s climate impact and quantified the climate mitigation potential of such operational improvements, aiming to identify new, more sustainable ways of flying.
Flying Low and Slow
The concept of flying low and slow consists of two linked processes. Flying on lower altitudes through warmer air masses reduces the probability of contrail formation, one of the dominating climate forcing processes, but the enhanced air resistance leads to a higher fuel consumption simultaneously. This excess of fuel burn can be compensated by a reduced cruise speed. The first part of the study about flying low and slow in ClimOP investigated the effects of reduced cruise altitude and speed, based on a daily sample of real flight track data of two widebody and two short-medium range aircrafts within the European airspace and beyond.
The selected air traffic sample covered 36% of the ECAC-area available seat kilometres (ASK) on the selected day. To quantify potential climate mitigation effects, flight routes were remodelled with flight performance models, realistic atmospheric reanalysis data, and the trajectory calculation module from DLR (TCM) and flight profiles of fuel burn and emissions were generated. Climate impact was derived in terms of Average Temperature Response aggregated over 20 years (ATR20) with algorithmic climate change functions (aCCFs), developed by DLR. In sensitivity studies, cruise altitudes were reduced in the flight trajectory simulations systematically by -2000ft, -4000ft and -6000ft, related to the reference flight level. In additional simulations cruise, Mach number was reduced by -5% and -10%. For all flights a European average seat load factor of 84% was assumed.
The results show an average climate mitigation potential of 6.3% for long-range flights and 12.5% for intra-ECAC flights, with respect to ATR20 allowing a mission-specific maximum fuel and time penalty of up to 5%. The average fuel increase is with 0.4%, and time enhancement of 1.3% by far lower to achieve a climate mitigation potential of in average 10% for both long and short-haul missions. For single flight missions, an ATR reduction of up to 64% is possible. The most efficient setup is on average a reduction of -2000ft cruise altitude and a speed reduction of -5%.
In a second sub-study, the seasonal effects were investigated. Therefore, one representative daily weather pattern per season over Europe was selected and combined with the corresponding daily air traffic sample and simulated with real atmospheric data. Deviating from the first part of the study, not all flights were considered for the analysis, and only the intersection of missions that were flown on all four selected days. Furthermore the trajectories between the airport city pairs were modelled along the respective great circle routes for all seasons to enable comparability. The seasonal analysis over 157 flights shows a higher climate mitigation potential in winter compared to summer due to a higher impact of contrails on the selected day.
An implementation of flying low and slow is linked to an enhanced air traffic controller workload, as the air traffic volume might be concentrated on few flight levels. Moreover, an extended travel time is directly connected to increased direct operating costs and may change the network integrity.
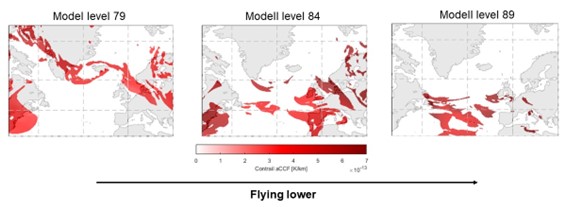
Change in contrail forming regions over the North Atlantic region with flight altitudes during night time for the selected summer day. Model level 79 represents an altitude of 34000ft, Model level 84 approximately 29500ft and Model level 89 approximately 24500ft.
Climate-optimised Intermediate Stop Operations
In order to reduce the effect of burning fuel for carrying fuel on long-haul flights, an intermediate stop for refuelling can be performed to reduce the aircraft’s weight. The Operational Improvement climate-optimised Intermediate Stop Operations (ISO) follows two aspects: a climate-optimised choice of the intermediate stop airport and the climate optimal cruise altitude instead of fuel optimal intermediate stop operations configuration. While the amount of CO2 emissions is directly linked to the fuel burn, the operational concept of climate-optimised ISO is mainly focused on the reduction of non-CO2 effects.
Regarding its climate mitigation potential, ClimOP’s investigation is based on a global annual flight plan for 2018 truncating missions with a great circle distance below 2500 nautical miles to make an application of intermediate stop operations useful.
This traffic sample guaranties a high coverage of the total annual ASK of all European long-range flights (97.7%). To select the appropriate intermediate stop airports, an eccentricity of 25% between the two legs were allowed, and an upper threshold of 20% total detour compared to the nonstop flight along the great circle was set. To keep the computational effort within a feasible limit, only the airport per region bordered by 30° degrees longitude and latitude grid box with the lowest detour was selected. The load factor was again assumed constantly for all flights with the European average of 84%. The model workflow was similar to the previous study. Flight profiles, performance and emissions were calculated with TCM. ATR20 and ATR100 was quantified for reference non-stop flights, and all selected ISO missions with the DLR model AirClim.
In this study, all trajectories were simulated along great circle routes, with both several constant cruise flight levels and fuel-optimal cruise altitudes performing step climbs in the course of cruise phase.
From the globally aggregated perspective, a climate-optimized intermediate stop can reduce the ATR by 39%, compared to a nonstop flight. This significant reduction in climate impact can mainly be explained by a reduction in contrail (- 52%) and NOx effects (- 49%), due to the changed routes, weights, and altitudes, while overcompensating an increase in CO2 effects of 18%, due to detours and less fuel-efficient flight levels.
It is necessary to keep in mind that additional take-offs and landings, due to application of climate-optimised ISO, increase both the pilot’s and the air traffic controller’s workload, and could exceed the capacity of the most popular ISO airports, with further effects on the entire network. The intermediate stop itself and the required time for refuelling both increases the travel time and the direct operation costs, related to additional airport landing fees, enhanced wear, reduced maintenance intervals, and enhanced costs for staff due to increased travel time.
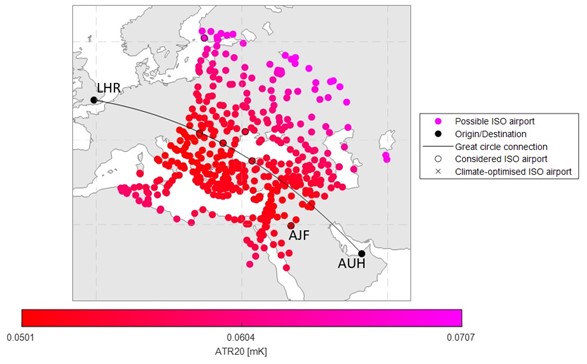
Location of possible intermediate stop airports and the associated ATR100 for an exemplary mission from Abu Dhabi to London with an A380 assuming optimal flight levels
Author: Christian Weder – DLR